Astronomers have long wondered how Earth became water-rich—bountiful with abyssal oceans, frigid glaciers and rain that pours from the sky into lakes, rivers and wetlands. Water, which is composed of the first and third most common elements in the universe, is a deceptively simple molecule to form. Yet while the details of its delivery to rocky planets like our own may be essential for understanding life’s cosmic prevalence, they remain mostly unknown.
Water is a potent medium for the assembly of complex organic molecules and offers havens for the emergence and subsequent evolution of life as we know it. Deep within Earth it ensures the lithic lubrication that keeps climate-stabilizing plate tectonics from grinding to a halt—another mechanism that just might be crucial for life. And when frozen as ice it plays a key role in planet formation by providing the glue that helps young worlds grow. As such, scientists are anxious to better understand water’s planetary peregrinations—the pathways it takes to transform planets from desiccated rocks into worlds as soaked as Earth.
To gain more insight, astronomers are utilizing the James Webb Space Telescope (JWST) to peer into protoplanetary disks: the swirls of gas and dust around young stars where planets are actively forming today. Although astronomers have glimpsed water within such disks before, their view has been hazy. For example, water vapor is visible to the Atacama Large Millimeter/submillimeter Array (ALMA)—in many respects the most powerful radio observatory on Earth—but the facility largely cannot detect water ice. This blocks a protoplanetary disk’s outer regions from ALMA’s scrutiny. The array also cannot deeply probe the hot, inner regions of disks where terrestrial bodies form. JWST, on the other hand, was designed with such studies in mind and has—quite literally—opened the floodgates. The new space observatory is delivering unprecedented looks at how water passes from star-forming giant molecular clouds into protoplanetary disks and finally onto planets—with significant implications for astrobiology, including whether our own watery world is somehow special or commonplace.
On supporting science journalism
If you’re enjoying this article, consider supporting our award-winning journalism by subscribing. By purchasing a subscription you are helping to ensure the future of impactful stories about the discoveries and ideas shaping our world today.
“With JWST it’s like suddenly you try on new glasses, and they give you a much sharper view,” says Andrea Banzatti, a Texas State University astronomer studying protoplanetary disks.
Water’s cosmic odyssey began hundreds of millions of years after the big bang. That’s when the first stars—which had furiously fused through their hydrogen store to churn out heavier elements—burst into supernovae that seeded the universe with oxygen. At this point, a single atom of oxygen could mingle with two hydrogen atoms to form a molecule of water—a molecule that could, in a cosmic cycle of creation and destruction, still be sundered anew by high-energy radiation from stars and other astrophysical sources. Yet sooner or later—undoubtedly billions of years later, in some cases—such water found its way to the cold confines of molecular clouds, where another chaotic and violent chapter of its journey would begin. Molecular clouds are giant, chilly clumps of dust and frozen gas that contain abundant water ice and serve as cradles for stars and planets alike. When part of a cloud reaches some critical density, gravity causes that dense, roughly spherical region to collapse into a flattened, whirling protoplanetary disk with a nascent, glowing star at its center. Much of this process is obscured by dust and has proved almost impossible to probe—until JWST. “It took JWST’s incredible sensitivity to enable us to catch the few photons that make it through and hence characterize the icy grains right before the onset of star and planet formation,” says Karin Öberg, an astronomer at Harvard University.
From there, the growing star feeds on material raining in from its enveloping disk, creating more light and heat—and potentially breaking apart the disk’s water molecules to bake off moisture that would have otherwise flowed into worlds. If this process happens too efficiently, the result would be galaxies full of parched planetary systems, and we might well not be here—which is in no small part why most scientists suspect this can’t be the case. Somehow water must pass unscathed from placid molecular clouds through sizzling star-forming disks.
In 2021 John Tobin, an astronomer at the National Radio Astronomy Observatory, and others used ALMA to observe V883 Orionis—a disk-wreathed protostar located 1,305 light-years from Earth that is only slightly more massive than our sun but shines roughly 200 times brighter. The protostar’s radiance heats up the frigid outer disk, transforming ice to water vapor that becomes a beacon for ALMA’s radio vision. It was a lucky break. Tobin’s team saw high ratios of semiheavy water—in which the heavier hydrogen isotope deuterium substitutes for one of a water molecule’s two standard, lighter hydrogen atoms. Because semiheavy water can only form in cold temperatures—and not the high temperatures directly associated with star formation—its origins around V883 Orionis must date back to the molecular cloud itself, suggesting that the water had passed through the star-formation process unchanged. Indeed, the ratio of semiheavy water to normal water that Tobin and his colleagues observed perfectly matched the proportion observed in other molecular clouds. It also matched the ratio found in our solar system’s comets—hinting at one way that water can reach rocky worlds.
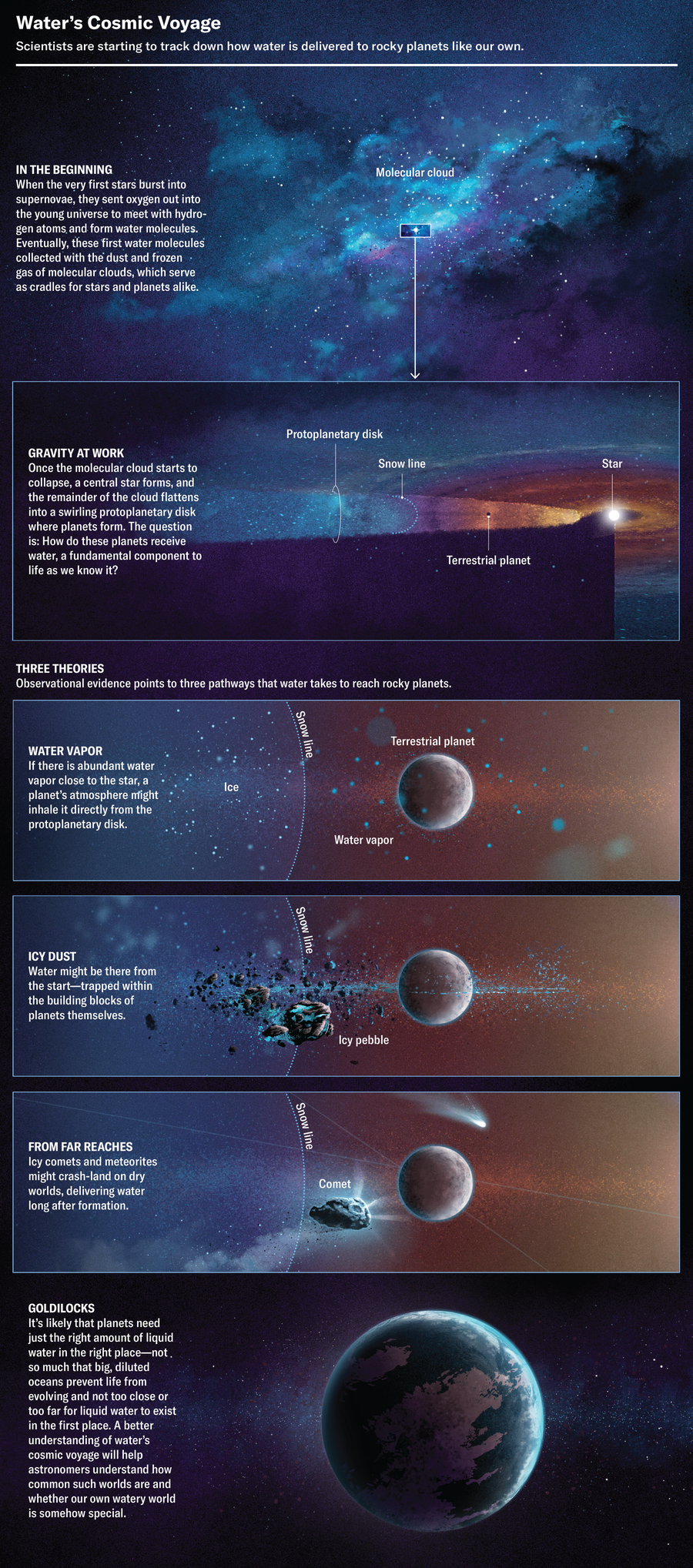
Astronomers currently think that terrestrial planets can gain water in three different ways. It could be that water is there from the start—cocooned within grains of dust that are the very building blocks of the planets themselves. Or perhaps the growing planets siphon water vapor directly from the gas in the primordial disk—allowing gravity to build a humid atmosphere around their rocky core. Or maybe once the planets have formed, they guzzle water imported via leftover icy debris falling in from far-flung regions of the planetary system. Tobin’s results suggest that this latter pathway plays a prominent role but that water-delivering comets and meteorites do not work alone. Water on Earth has a slightly lower ratio of semiheavy water to normal water than that found in comets—meaning that while much of our planet’s water arrived from the outer solar system’s icy hinterlands, some must have instead been exposed to high temperatures near the sun. Just what that exposure looks like, however, remains an open question.
To find answers, Öberg wants to build a cosmic map—pinpointing the locations of water around these young protoplanetary disks to see where it is readily available to feed any forming worlds. ALMA has already sketched a blurry image, and JWST is beginning to fill in the gaps with spectacular detail. Last April Sierra Grant, an astronomer at the Max Planck Institute for Extraterrestrial Physics in Garching, Germany, and her colleagues used JWST to observe water in a disk that had previously appeared dry—demonstrating the space observatory’s remarkable capability to wring fresh insights even from well-studied systems. “We are really in a new era with JWST,” Grant says. “It’s remarkable that we could detect things that we couldn’t detect before.”
Then last August Giulia Perotti, an astronomer from the Max Planck Institute for Astronomy in Heidelberg, Germany, and others detected water vapor in PDS 70—the sole protoplanetary disk yet known to harbor not one but two giant planets. Their presence likely means that terrestrial planets are also coalescing within the inner disk—at the precise location where astronomers have now found water. “This is the first time we are detecting water vapor in the central regions of a planet-hosting disk,” Perotti says. Previous observations had shown no water at all—which was unsurprising, given that water would be hard-pressed to survive the intense radiation so close to the star. Now astronomers know that it can and that it may just glom on to the atmospheres of planets as they grow—as opposed to reaching dry planets via interplanetary visitors later on. As such, most terrestrial worlds across the cosmos might be born rich, with a wealth of water from the very start.
That by itself could be a problem, Öberg says, that could lead to worlds that are too water-rich. Scientists currently think that ocean worlds will struggle to create life, but a planet with pond-peppered continents will have much better luck. That’s because many of the reactions thought crucial for prebiotic chemistry and the rise of complex chemical systems proceed much more efficiently in small, concentrated ponds than in expansive, diluted oceans. Moreover, minerals that erode from the continents will add crucial nutrients to the water. But to learn how often the universe builds terraqueous orbs like Earth, Öberg argues that we first need to understand young protoplanetary disks—by completing that cosmic map, which shows not just the locations of water around these disks but also how it flows from one locale to the next.
Just how water pours from the icy outer region of the disk inward, however, has been unclear—especially in PDS 70, where there is a large gap between the outer and inner disks. Then last November Banzatti, Öberg and others observed water vapor within the system’s snow line, the transitional region where shifting temperatures transform water from solid to liquid or gas. This confirmed a physical process whereby water migrates inward. Forty years ago, astronomers postulated that water ice in the outer disk would drift inward on sturdier solid material—dust grains and so-called icy pebbles that range in size from a few millimeters up to a few meters—until sublimating at the snowline in a great fog of water vapor. That’s the precise signature that Banzatti observed. “This is exactly what was expected in this fundamental theory of planet formation, this ‘pebble drift’ scenario that should feed the formation of [terrestrial planets] and even deliver water,” Banzatti says. “From that little signal we can build a beautiful story.” And it has implications for PDS 70. Perotti suspects that this mechanism carried water inward before the gap formed between the outer and inner regions of the system’s disk. Or it could be that water continues to migrate through the gap even now, albeit on much smaller as-yet-unseen micron-sized icy dust. This dust, along with some water molecules, can act as a protective shield, keeping many water molecules from breaking apart.
Öberg’s cosmic map is thus becoming more and more detailed—with vast reservoirs found in many regions of protoplanetary disks, suggesting that water may well flow to rocky worlds in myriad ways. But still, astronomers do not know if one scenario has the upper hand. “At this stage of our research, we are not in the position of being exclusive,” Perotti says. In PDS 70, for example, it could be that these terrestrial planets lose some of the water that is readily available to them—relying on asteroids to later replenish it. Future observations should shed light on the dominant pathway, which might change depending on certain characteristics of the planetary system.
Grant, for example, is eager to see how water’s dynamics scale with stellar mass. Thus far, high-mass stars appear to be mostly dry⁠ whereas smaller, more sunlike stars appear to be relatively waterlogged, but Grant wants to know what is typical for some of the most diminutive stars. Called M dwarfs, these stars are faint, meaning that planets circling them must be in a close orbit to be warm enough for life—a quirk that makes them relatively easy for planet hunters to investigate using JWST and other telescopes. Their protoplanetary disk has long appeared water-poor—but fresh data now suggest otherwise. Last December Banzatti, Öberg and their colleagues published a study detailing the first case of a water-rich disk around an M dwarf star. Grant plans to probe this question further by exploring as many small stars as she can. Meanwhile Banzatti’s ongoing analysis of snowlines from 30 different systems is already revealing that small, compact disks send 10 times more water toward their inner regions than large, extended, gap-filled disks can manage. Water’s cosmic journey is, at last, coming into focus.
“It’s really exciting to see these results coming together,” Tobin says. “It’s an astounding time of discovery—yet we’ve really only scratched the surface of what’s out there.”