JWST Solves Decades-Old Mystery of Nearby Supernova
Scientists have finally found the compact object at the heart of the famous supernova of 1987, and it’s not a black hole
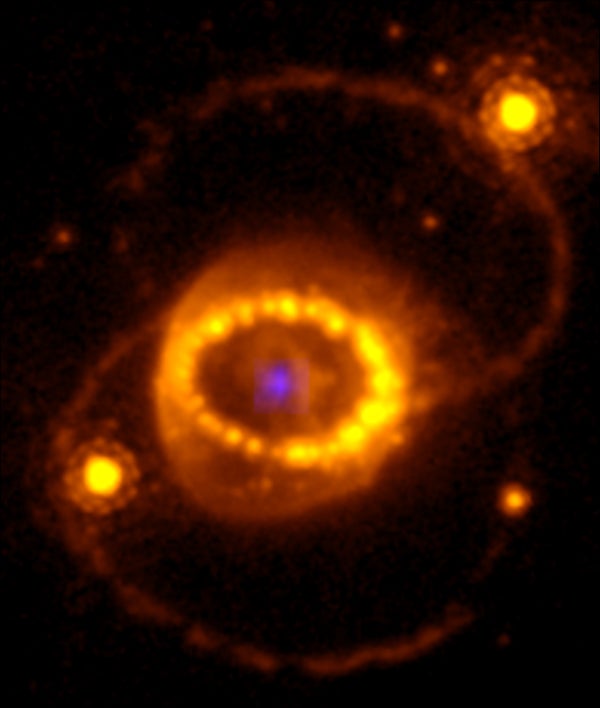
Nearly forty years ago residents of Earth were treated to a rare cosmic sight: an exploding star in our sky that was visible to the naked eye. Called Supernova 1987A (SN 1987A), it was the closest such event of the past four centuries. Ever since astronomers have sought to observe the stellar remnant that they have known must lurk near the supernova’s center, shrouded within an expanding nebula of radioactive ash and incandescent gas. Now, thanks to the power of the James Webb Space Telescope (JWST), a team of scientists has finally found that elusive quarry, confirming suspicions that the explosion created an extremely dense neutron star rather than a starlight-swallowing black hole.
The discovery, published on Thursday in Science, used JWST’s unprecedented infrared capabilities to pierce the veil surrounding SN 1987A, allowing it to be seen in a literal new light. Peering into the heart of the debris left behind by the star’s demise, astronomers led by Claes Fransson of Stockholm University in Sweden spied hints of ionized argon and sulfur—that is, evidence of elements that were so shocked by some external force that their electrons had been stripped away. These energized elements would not be expected to exist so close to SN 1987A’s “ground zero”—unless they formed from intense ultraviolet and x-ray bombardment from a nearby neutron star. A feeding black hole burping out blasts of radiation could also explain the result, but more than three decades of observations have failed to reveal any other signs of such a thing within SN 1987A, making JWST’s result almost airtight proof of the neutron star’s existence.
“It’s very exciting,” says astrophysicist Mikako Matsuura of Cardiff University in Wales, who was not involved with the study and previously suggested in 2019 that a neutron star would be found in this supernova. “It’s probably the strongest evidence of the presence of a neutron star in Supernova 1987A.”
On supporting science journalism
If you’re enjoying this article, consider supporting our award-winning journalism by subscribing. By purchasing a subscription you are helping to ensure the future of impactful stories about the discoveries and ideas shaping our world today.
SN 1987A exploded on February 23, 1987, in the Large Magellanic Cloud, a dwarf satellite galaxy of the Milky Way that is about 160,000 light-years from Earth. No supernova had been seen so close to our planet since Kepler’s Supernova in 1604, when a star detonated within our galaxy at a distance of about 20,000 light-years. Although SN 1987A was initially discovered via its sudden brightening in the sky, the first sign of the supernova proved to have come from a burst of neutrinos that washed over Earth a few hours before the flash of light. Recorded in neutrino observatories scattered across the planet, that burst was telltale evidence of a neutron star’s formation somewhere within the star’s scattered remains. The case for a neutron star grew as further analysis revealed SN 1987A’s progenitor had likely been a blue supergiant star about 18 times the mass of our sun—heavy but still too light to easily form a black hole.
Supernovae occur in two main ways: One is when a star siphons too much material from a smaller companion star and explodes—this results in a type Ia supernova such as Kepler’s Supernova. The other kind of supernova—a type II supernova such as SN 1987A—occurs when a very massive star that has been kept from collapsing under its own weight by the outward pressure of light blasting out from its depths suddenly runs out of fuel in its core. With no surplus of starlight to support it, the star’s outer layers fall inward to the core and then rebound to explode outward, sending shockwaves rippling through the surrounding material. This process can rapidly emit more light than an entire galaxy’s worth of stars and crushes the solar-mass core into a city-sized ultradense sphere—a neutron star. In cases where the initial star was especially hefty—20 solar masses or more—the heavier resulting neutron star then collapses into a black hole.
Having a neutron star so relatively nearby is scientifically fascinating, says Joanne Pledger of the University of Central Lancashire in England, who was not involved with the study. “Physics is different on a neutron star,” she says, noting that these objects’ extreme gravitational fields squeeze their innards to create exotic states of matter and significantly warp the surrounding fabric of spacetime. “If we can detect neutron stars, particularly close neutron stars we can study very well, then we can start to understand the laws of physics in areas we just can’t re-create in a lab.”
Although astronomers already suspected SN 1987A had not left behind a black hole, they wanted to be sure. Fransson and his colleagues spied the distinctive signs of ionized argon and sulfur conspicuously close to the supernova’s center in July 2022, when JWST was first beginning its science operations. “[SN 1987A] was one of the first objects observed,” Fransson says, with JWST studying the supernova’s aftermath for about 10 hours.
“The only energy source capable of producing those [signs] is a neutron star,” says study co-author Patrick Kavanagh of Maynooth University in Ireland. For a black hole to do the same, it would need to be ravenously feeding on matter from a source—such as another star—for which there is no evidence. “We’re confident that we assessed all the different possibilities,” Kavanagh says. “We ruled out everything except the presence of a neutron star.”
Carefully parsing the light emitted by the ionized material shows that the neutron star is not exactly at the middle of SN 1987A; rather, it is slightly offset because it received a “kick” from the supernova. As the star exploded, any minor imbalances would have shifted more of the outpouring matter to one side or another, causing the neutron star to recoil in the opposite direction like an egg squeezed from a balloon. Observations suggest the neutron star is moving slightly toward us, having traveled about 500 billion kilometers from the site of its cataclysmic birth. “The kick velocity is around 400 kilometers a second,” Kavanagh says—unthinkably fast for us here on Earth but still glacially slow against the immensities of light-years.
What’s not clear is whether SN 1987A’s remnant is just a neutron star. Instead it might be a pulsar—a neutron star spinning so rapidly that it fires streams of energy from its poles that sweep across a swath of sky like the beams of a cosmic lighthouse. “If there is a pulsar, the beam is not pointed at us, so we can’t detect it,” says Yvette Cendes of the Center for Astrophysics | Harvard & Smithsonian, who was not involved in the study. But there might be another way to find out. In the standard neutron star scenario, the sheer heat from the stellar remnant is so intense that it forms a beacon of ionized silicon that is scattered farther away in the expanding cloud. In the pulsar model—where emissions aren’t dominated by heat but rather by winds of electrons and other particles that shock the innermost debris—ionized silicon should be more scarce. So if silicon can be spotted and mapped around SN 1987A, “we can discern between the two,” Kavanagh says. As yet unpublished follow-up JWST observations that were taken by the team in the fall of 2023 and earlier this week may contain that answer.
These observations offer fresh insights into the earliest moments after a type II supernova. “We haven’t seen the formation of a neutron star before,” Fransson says. Now that we have, further studies of this cosmically young object with JWST and other telescopes should allow astronomers to learn much more about these bewildering stellar events. “Until we see a supernova in our own galaxy, this is the best studied one we’re going to have,” Cendes says.